It’s just around the cornea! How a new 3D model could help find effective treatments for blindness.
By LifETIME CDT Student: Victoria Hughes (University of Birmingham)
Losing my senses is one of my biggest fears, and at the top of that list would be losing my sight – a tough reality that affects up to 250 million people worldwide. Most blindness in the world is preventable and can be attributed to a lack of available and/or affordable eye care. Whilst treatments are available for several causes of blindness, for example refractive errors (needing glasses) or cataracts (corrected with surgery), the fourth leading cause of blindness is not so easily treated – this is corneal blindness. The cornea is the transparent front of the eye which acts as a window for light to pass to the retina, allowing us to see. Injury/disease can cause scarring to the cornea, which affects the admittance of light through the eye and impairs vision irreversibly. Currently, the only curative treatment for blindness of the cornea is a corneal graft; this requires a healthy cornea, taken from a deceased human donor, which can be stitched into the recipient’s eye to replace their diseased one. However, there are 2 major problems with corneal grafts; the high risk of immune rejection, and the lack of available donor tissue (only 1 cornea is available for every 70 needed!). Clearly, there is a desperate need for more effective treatments that can help reverse the effects of corneal blindness and restore a patient’s vision. Complicating things further, there are a lack of representative models of the cornea that can be used in labs to test treatments. You may be asking, but what is a model? Let me explain: A model is a representation of something. You may be familiar with the concept of train models; basically, a small version of a real machine. Scientific models are similar: we can take our knowledge of the biology and functions of a tissue or organ and make a version of it in vitro (in a lab) that mimics the real thing.
This is where my project comes in; I want to build a 3D model of the human cornea that mimics the biological functions and mechanical structures of real corneal tissue. It will look something like the image in Figure 1. So how do I plan to do this? There are various aspects to my project which I will highlight below.
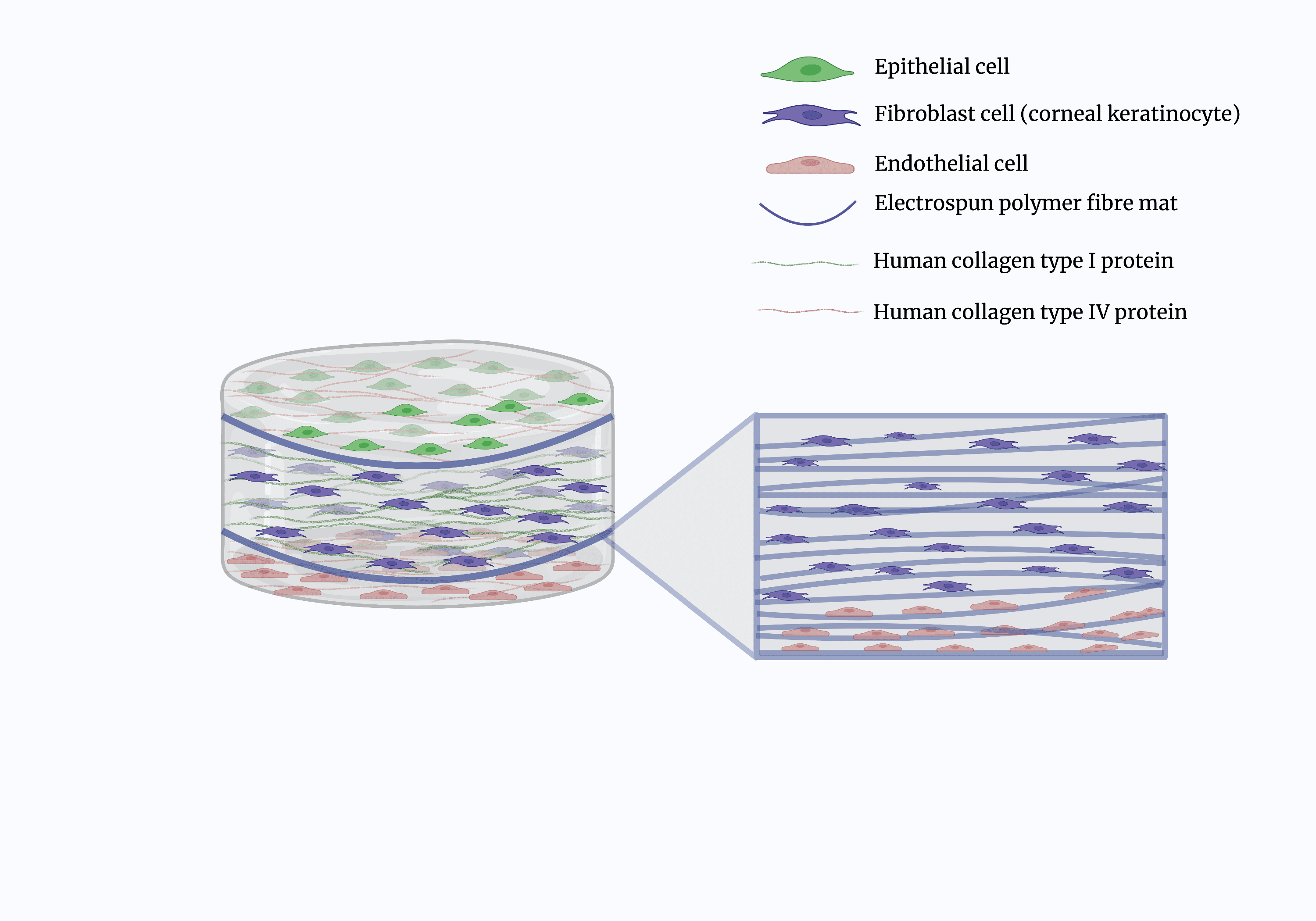
Fig. 1. Schematic representation of corneal model, showing the various cell types, collagens, and polymer mats.
Cell culture
Perhaps the most important aspect of my model is incorporating human corneal cells. Referring to Figure 2 as guidance, you can see there are 3 distinct cell types in a human cornea; epithelial cells (cells of the outermost layer of the cornea); corneal keratinocytes (these populate the middle and largest layer of the cornea); and corneal endothelial cells (these exist in the deepest layer of the cornea). I plan to culture all 3 cell types and grow them into the relevant layers of my model.
Hydrogels
Hydrogels are mostly water blended with a molecule, often polymers (think of a plastic) or peptides (parts of a protein) to create a jelly-like substance. These materials reading attract water molecules but do not dissolve in water, making them desirable for use as implantable or injectable devices – bodies contain a lot of water! They are also cell-friendly and able to incorporate extracellular matrix (ECM) components, which are different molecules needed to help cells grow. For this reason, hydrogels are frequently used as building blocks for tissue models. In my project, I blend different types of collagen protein into my hydrogels to create distinct layers that will replicate the biochemical structure of a real human cornea – also encouraging cells to deposit their own ECMs and grow in the correct orientation.
Electrospinning
This is a process by which a polymer solution (a type of plastic dissolved into a solution) is extruded through a needle and arranged into fibres by attracting to a voltage. For my experiments, the fibres are collected onto a mandrel which spins at a high speed to encourage the fibres to align. Over an extended period, fibres will stack on top of each other and build a mat. Many types of polymers exist, but some have been of particular interest to tissue engineering projects as they have biocompatible characteristics; this means they are not harmful to cells. I plan to use these polymer mats
as a support structure upon which I will print the hydrogels; this way, I can define separate layers like in a real cornea. The electrospun mats will also act as a physical support to reduce the likelihood of the gels collapsing.
In conclusion, my project is aiming to fill a gap in the literature by building a full thickness model of the human cornea. I hope this will serve as a tool for drugs to best tested on, to predict how a real human cornea would respond to them. Stay tuned to find out how this goes over the next 4 years!