Rub-a-dub-dub cell sensors in the tub: monitoring cell therapies in bioreactors
By LifETIME CDT Student: Hannah Williamson (University of Birmingham)
Cell therapies, medicines made from human cells, have had huge success in fighting leaukemias and offer new hope to patients as treatments for a range of diseases. However, the manufacture of these therapies remains a major challenge and barrier to patient access. New technologies are therefore needed to develop reliable and robust manufacturing processes which include continuous quality control monitoring… enter the need for on-demand sensing of cell therapy culture!
In cell therapy manufacture, cells are taken from individual patients, modified and then grown in containers called bioreactors before being purified and infused back into the patient. As humans we are all different, therefore it is unsurprising that there is increased variability and unpredictability when growing individual patient cells compared to the commercialised, well characterised cells used in research. But just like other medicines, cell therapies are required to meet certain critical quality attributes (CQAs) to ensure product identity and potency. Process parameters also need to be monitored and controlled to attempt to reduce product variation, to ensure safety and ultimately make what you want to make (in part, your process is the product!).
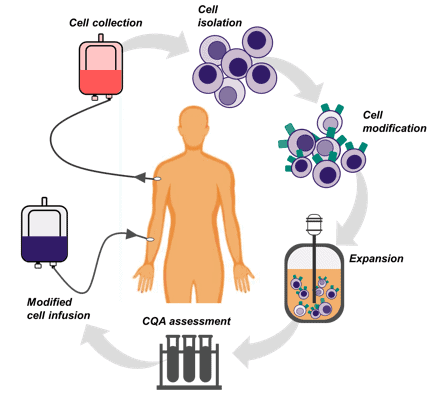
Figure 1
However, CQAs are monitored off-line using labour intensive, time consuming methods usually at the end of culture periods which doesn’t allow for production control. Furthermore, the parameters that are currently measured such as pH, temperature and glucose levels only provide us with a basic understanding of the conditions in the bioreactor and cell “happiness”. Monitoring protein marker cell attributes and those released by cells during cell culture would provide insights throughout the expansion phase.
For some cell types, proteins are also added to the bioreactor and are crucial in influencing cells to grow into the right product. These proteins are expensive and are currently added regimentally and sometimes needlessly when levels may not be low because levels are not measured. This increases the cost of culture, which is a main cost driver in the manufacture of cell therapies and ultimately affects the final pricing of these medicines.
(And in the range of $300-500,000, these prices are high!)
Measuring the levels of these supplemented proteins would therefore inform supplementation regimes and hopefully reduce needless spending in cell therapy manufacture.
There is currently no multi-marker continuous monitoring of proteins in cell therapy culture despite the need. My project therefore aims to address this by developing a biosensor able to detect multiple proteins, that can be integrated into bioreactors.
They might sound futuristic but biosensors have been knocking around since the 1970s. Just like how a metal detector is programmed to detect metal, biosensors are programmed to detect specific analytes. This is achieved through the use of capture elements which recognise the analytes of interest and bind them. Binding events are then translated into signals, electrical or optical, with more binding events causing larger signals. We aim to use an electrochemical technique called electrochemical impedance spectroscopy (EIS) to detect analyte binding to Nanobodies, our recognition element of choice. More analyte binding to the nanobodies immobilised on an electrode means there are more molecules at the electrode surface preventing electron movement. Imagine trying to move through a crowd of people compared to an empty street. It will be much easier to walk along the empty street but in the crowd, it’ll be more difficult and you might end up stuck. This is a similar case to electrons trying to move across surfaces where there is a high concentration of analytes bound. Higher binding of analytes therefore means electron movement is more hindered leading to an increase in capacitance which we can detect using EIS.
But this biosensor is a little different compared to standard electrochemical biosensors… we’re switching it up (literally). We are aiming to incorporate switchable surface technology, which has been developed in the Mendes lab for the past decade, to electrically control the binding of analytes to Nanobodies. Hopefully, this will allow us to switch the biosensor surface from an “OFF” state to an “ON” state, making the sensor capable of on-demand sensing of analytes.
With this technology we aim to fill a much-needed gap in cell therapy manufacture and switch up the game.
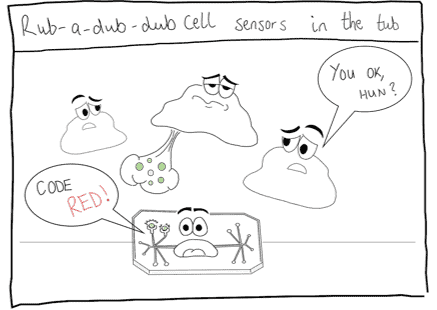
Figure 2